Reaction Energetics
Chemical reactions involve breaking and making some (or even all) of the bonds that hold together the atoms of reactant and product molecules. Energy is required to break bonds, and since the strengths of different kinds of bonds differ, there is often a significant overall energy change in the course of a reaction.
In the combustion of methane, for example, all six bonds in the reactant molecules are broken, and six new bonds are formed in the product molecules (equation 1).

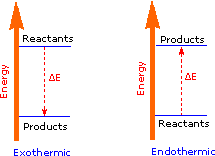 The sum of the product bond strengths in this case is greater than the sum of reactant bond strengths; consequently, the products are energetically (or thermodynamically) more stable than the reactants, and energy is released in the form of heat. Such reactions are called exothermic.
It is helpful to think of exothermic reactions as proceeding from a higher energy (less stable) reactant state to a lower energy (more stable) product state, as shown in the diagram on the right.
Reactions in which the products are higher energy than the reactants require an energy input to occur, and are called endothermic. Photosynthesis (equation 2) is an important example of an endothermic process. Energy in the form of photons (sunlight) drives the reaction, which requires chlorophyll as a catalyst.

Common sense suggests that molecules in which the bonds are all strong will be more stable than molecules having weaker bonds. Previously we defined bond strengths as the energy required to break a bond into neutral fragments (radicals or atoms).
The sum of all the bond energies of a molecule can therefore be considered its atomization energy, i.e. the energy required to break the molecule completely into its component atoms. If this concept is applied to a group of isomers, it should be clear that all the isomers will have a common atomization state, and that the total bond energy of each isomer is inversely related to that isomer's potential energy.
Thus, that isomer having the greatest total bond energy has the lowest potential energy and is thermodynamically most stable. To summarize, bond energy is energy that must be introduced to break a bond, and is not a component of a molecule's potential energy.
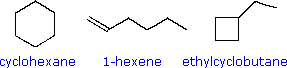 The three C6H12 isomers on the right illustrate this relationship. Cyclohexane is made up of six C-C sigma bonds and twelve C-H sigma bonds configured in a strain-free six-membered ring.
The isomer having a double bond, 1-hexene, on the other hand, has four C-C single bonds (all sigma) and one C-C double bond (one sigma and one pi bond). Since the pi bond is weaker than a sigma bond, cyclohexane has a larger total bond energy (by nearly 20 kcal/mol) and is thermodynamically more stable than 1-hexene.
The four-membered ring compound, ethylcyclobutane, has the same kinds of bonds as cyclohexane, but they are weakened by ring strain to such a degree that this isomer is even less stable (thermodynamically) than 1-hexene.
1. Activation Energy
Since exothermic reactions are energetically (thermodynamically) favored, a careless thinker might conclude that all such reactions will proceed spontaneously to their products.
Were this true, no life would exist on Earth, because the numerous carbon compounds that are present in and essential to all living organisms would spontaneously combust in the presence of oxygen to give carbon dioxide-a more stable carbon compound.
The combustion of methane (eq.1), for example, does not occur spontaneously, but requires an initiating energy in the form of a spark or flame. The flaw in this careless reasoning is that we have focused only on the initial (reactant) and final (product) states of reactions.
To understand why some reactions occur readily (almost spontaneously), whereas other reactions are slow, even to the point of being unobservable, we need to consider the intermediate stages of reactions.
Every reaction in which bonds are broken will have a high energy transition state that must be reached before products can form. In order for the reactants to reach this transition state, energy must be supplied and reactant molecules must orient themselves in a suitable fashion.
The energy needed to raise the reactants to the transition state energy level is called the activation energy, ΔE‡. An example of a single-step exothermic reaction profile is shown on the left above, and a similar single-step profile for an endothermic reaction is in the center. The activation energy is drawn in red in each case, and the overall energy change (ΔE) is in green.
The profile becomes more complex when a multi-step reaction path is described. An example of a two-step reaction proceeding by way of a high energy intermediate is shown on the right above.
Here there are two transition states, each with its own activation energy. The overall activation energy is the difference in energy between the reactant state and the highest energy transition state. We see now why the rate of a reaction may not correlate with its overall energy change.
In the exothermic diagram on the left, a significant activation energy must be provided to initiate the reaction. Since the reaction is strongly exothermic, it will probably generate enough heat to keep going as long as reactants remain.
The endothermic reaction in the center has a similar activation energy, but heat will have to be supplied continuously for the reaction to proceed to completion.
Virtual Textbookの目次へ |